Outer space is not exactly a friendly environment, which is why we go through great lengths before we boost people up there. Once you get a few hundred kilometers away from our beloved rocky planet things get uncomfortable due to the lack of oxygen, extreme cold, and high doses of radiation.
Especially the latter poses a great challenge for long-term space travel, and so people are working on various concepts to protect astronauts’ DNA from being smashed by cosmic rays. This has become ever more salient as NASA contemplates future manned missions to the Moon and Mars. So let’s learn more about the dangers posed by galactic cosmic rays and solar flares.
Radiation from Space
When German Jesuit priest and physicist Theodor Wulf climbed the Eiffel Tower in 1910 with an electrometer he wanted to show that natural sources of ionizing radiation are originating from the ground. And while readings on top of the tower were lower than the ground level, they were still much higher than would be expected if the ground were the only source. A few years later, Victor Hess undertook several risky balloon flights up to altitudes of 5.3 km to systematically measure the radiation level by observing the discharge rate of an electroscope. His experiments showed that radiation levels increase above ~1 km, and he correctly concluded that there must be some source of radiation penetrating the atmosphere from outer space.
The cosmic rays that rain down on Earth are predominantly protons (~90%), helium nuclei (~9%), and electrons (~1%). In addition, there is a small fraction of heavier nuclei and a tiny whiff of antimatter. These particles are blasted to us from the Sun and other galactic and extragalactic natural particle accelerators like supernovae or black holes.
How Earth Keeps Us Safe
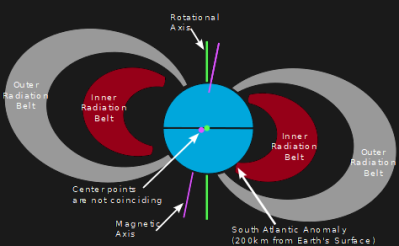
Credit: Marko Markovic, public domain
Luckily for us, the Earth’s magnetic field deflects many of the charged particles so that they never hit the atmosphere. Some of them are captured in two donut-shaped rings around the Earth, the Van Allen Belts. In addition, the atmosphere also does a good job of protecting us. Primary cosmic rays hitting the atoms in our atmosphere produce a shower of secondary particles with much lower energies so that most of them will not reach the ground.
The average radiation exposure here on Earth is about 3 mSv per year. Depending on your altitude, about 0.39 mSv (13%) is contributed by cosmic radiation. Flying in an airplane at 12 km altitude increases your radiation exposure about 10x compared to sea-level. Things are even worse if your flight route is near the geomagnetic poles where charged particles are concentrated. If you are interested in the radiation dose you will receive on your next flight, check out NASA’s NAIRAS webpage. It provides a global map of radiation dose rates for different altitudes that are produced in near real-time from measurements of solar activity and cosmic rays.
Doses on the ISS, the Moon, and Mars
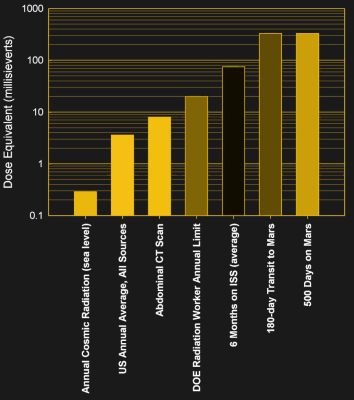
Credit: ASA/JPL-Caltech/SwRI, Public Domain
For a crew member of the ISS, the average radiation dose is about 72 mSv for a six-month stay during solar maximum: it is about 50 times higher than on Earth. Because the contribution from solar particles varies with the 11-year solar cycle, one might think that the radiation dose is lower during solar minimum. But in fact, the dose is about twice as high as during solar maximum.
This is because the dominant source of radiation on the ISS is galactic cosmic rays (GCR), which are deflected by the Sun’s magnetic field. GCR intensity is highest during solar minimum when the Sun’s magnetic field is weakest. Even a big solar particle event (SPE) like a solar flare or coronal mass ejection cannot hurt ISS astronauts much but instead sweeps away other charged particles thereby reducing the GCR flux for weeks. This effect is known as the Forbush decrease.
Another big contribution to the radiation exposure aboard the ISS comes from protons trapped in the Van Allen belt. The exposure is largest when the ISS crosses the so-called South Atlantic Anomaly (SAA) where the radiation belt comes closer to Earth’s surface. Most of the time, the ISS orbits comfortably inside the Van Allen belt, but during the 5% of the mission time that the ISS is spends in the SAA, it can be responsible for more than 50% of the total absorbed radiation dose.
The Moon, on the other hand, is exposed to all kinds of cosmic rays since it is located much further away and has neither a magnetic field nor an atmosphere. Here SPEs like the big solar storm that occurred in August 1972, shortly in between the Apollo 16 and Apollo 17 lunar missions, can be life-threatening events for astronauts. Recently, the Lunar Lander Neutrons and Dosimetry (LND) experiment aboard China’s Chang’E 4 lander has measured the radiation exposure on the lunar surface. With about 500 mSv/year the radiation dose is nearly 200 times higher than on Earth.
Plans for manned missions to Mars are already underway and radiation exposure is one of the main concerns. Estimations of the radiation dose for a trip to Mars are based on measurements of the Radiation Assessment Detector (RAD) piggybacking on the Curiosity rover. During a 360-day round trip, an astronaut would receive a dose of about 660 mSv. Assuming that the crew would spend 18 months on the surface while they wait for the planets to realign, they will be exposed to an additional 330 mSv. So the total exposure for an astronaut going to Mars and back will be around 1,000 mSv. This is equal to the exposure limit for NASA astronauts during their entire career, and corresponds to a 5.5% risk of death due to radiation-induced cancer. Therefore, although there is certainly a severe health risk, radiation exposure is not a showstopper for a mission to Mars.
Protecting Space Travelers
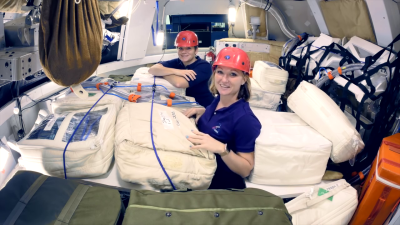
Credit: NASA
Astronauts aboard the ISS are partially protected against radiation by the station’s aluminum hull. Materials with low atomic number provide the most effective protection against space radiation including secondary particles like neutrons. Such lightweight materials are also cheaper to bring into space. The ISS has equipped their crew quarters with polyethylene bricks that reduce the radiation dose by 20%.
If we ever construct settlements on the Moon, they will probably be buried beneath lunar soil to be shielded from SPEs and other cosmic rays. On the downside, this would also increase the exposure to secondary neutrons, as measurements of the Apollo 17 Lunar Neutron Probe Experiment have shown.
NASA’s Orion spacecraft is planning to bring people to the Moon in 2024 and eventually to Mars. Since the additional mass for dedicated shielding would simply be too expensive their radiation protection plans include the possibility to build a shelter from onboard supplies including food and water in case of an SPE.
Let’s not forget that space radiation is not only dangerous to humans but also to electronic equipment. High-energy particle interactions can easily damage critical electronic systems on a spacecraft and are causing total or partial mission loss for between one and two satellites per year. Extreme SPEs can even influence equipment on Earth like the 1972 solar storm which is said to have caused the accidental detonation of two dozen US sea mines in Vietnam.
For the future of space travel, more advanced active shielding concepts are the topic of ongoing research. These include powerful magnetic or electrostatic fields and plasma “bubbles” surrounding a spacecraft to deflect charged particles. Of course, radiation protection is only one of the many challenges posed by long-term space travel but let’s hope we will find ways to keep our astronauts safe and healthy.
Solar flare? Build a pillow fort!
And then it dawns on you that our atmospheric pressure of 100 kPa means there is ten tonnes of air per square meter on top of us…
‘Cause I am barely breathing
And its ten tonnes of air
Don’t know who I’m kidding
Imagining you care
Factoid of Interest! One atmosphere can support 10 meters of Lunar Regolith without structural help. Huge well shielded spaces are possible on the Moon with very little building material.
“On top” only applies for perpendicular angles, if the average angle of cosmic ray arrival is (say) 45 degrees to straight down then you get an extra √ 2 = 1.4 factor – so 14 tonnes worth of shielding.
Is this not why we assume a spherical cow surrounded by a spherical shield of uniform thickness.
Perhaps the answer may be medical. If we can find ways to help our bodies neutralize the effects of radiation, it may be easier than trying to shield ourselves from it.
So, like Niven’s Bandersnatch. Too bad for the remaining humans.
Or, as a side effect, these medical knowledge could lead to an eradication of cancer? That would also help the humans which remain on earth. Otherwise such research should not harm them it should no
Just give’m some Hyronalin and they’ll be fine.
Plus Lectrazine.
Hmmm. Should the ships use Dimonium or Diamondinium?
We could use Chernobyl liquidators to breed a new generation of radiation-resistant humans designed for space use.
Hmm, well, better auto-repair systems were what I had in mind, or better cures for cancer.
It’s more likely that you spotted a Latina.
Mate I’m married to a latina, so nope, she was a very dark brown caucasian, and I know for a fact that there is a drug that has that effect, first trials in Australia were as much as two decades ago. I always wondered what came from that research.
Few years ago you could buy a tanning kit online, an injection that makes you darker. It was quite controversial and then dropped off the radar. This is probably what became of it
One might want to go to the SPENVIS sight, which will model dose for a given orbit or trajectory.
https://www.spenvis.oma.be/intro.php
It uses a variety of base models including CREME96 (and others) for Galactic Cosmic Rays, and a variety of solar proton models such as King, JPL, and ESP. Other models are used for heavy ions.
Can we use similar method like Earth. If create magnetic field, wouldn’t this protect the astronauts?
Yes, that can be one component of an overall shielding strategy. The problem is not the shield end of things but the powerplant required for such active measures. Once we have compact fusion reactors offworld then we can start doing some really funky things like that. Imagine a crew shelter that is like the core of a fusion reactor, that toroidal space could be the shielded area, and use very similar superconducting magnet tech to the powerplant. Make it really big and spin it then you have the entire habitable area of the ship or station shielded and with pseudo-gravity.
I wonder how strong of a field is required. Could it be generated by rare earth magnets and some kind of pole pieces around the crew quarters?
The field needs to big rather than strong. Some of these particles fly at very high speeds, so if you want to bend their path at the last moment, you would need insanely high field strength.
If your field is bigger, you can give the particles a more gradual push.
A magnetic field only affects charged particles (Cosmic Rays) and it can turn them, not stop them or reduce their energy (The force is a cross-product. It can not do work.) They can also turn Solar Wind, but that energy is trivial.
Cosmic rays are photons (gamma, x-rays, etc.) and not charged, so magnetic fields don’t do anything) The only thing that helps is mass
No, “cosmic rays” are overwhelmingly charged particles. Protons and charged nuclei.
Yes, you’re right, I misremembered
I get the “romance” of space exploration, but I’ve long wondered about Polyanna public attitudes toward radiation injury (especially by prejudiced interests, on Earth as well as in space.) Does the hefty 1.0 Sv estimate for a Mars round trip include a term for occasional (“life-threatening”) SPEs or is it an optimistic average, which glosses over the chance of serious “sun burn”? On the positive side when staying on a substantially unshielded extraterrestrial body like Mars can’t subsurface shelters be used to limit radiation doses (as on the Moon?)
Why would you want to go all the way to Mars, only to live inside a shelter ? You can live underground right here on Earth, for a lot less trouble.
+1
Yes. I don’t think human exploration into deep space (further than the moon) is feasible in the foreseeable future. Robots are continually getting cleverer; humans are not getting tougher.
M.O.T.
(Massively Off Topic)
Yeah for the immediate future, you probably don’t want to do a long duration mission in your 20s or 30s. Save it for your 40s or 50s.
But realistically, we have the technology to reduce the amount of radiation reaching Mars: https://phys.org/news/2017-03-nasa-magnetic-shield-mars-atmosphere.html
IMO, we can deploy something like this (about the size of a large satellite) and significantly reduce the radiation exposure on Mars’ surface.
Photo caption: “The radiation protection plan for the Orion spacecraft includes building a shelter from stowage bags during an SEP event.”
Why should I worry?
It is clearly Someone Else’s Problem.
B^)
“High-energy particle interactions can easily damage critical electronic systems on a spacecraft and are causing total or partial mission loss for between one and two satellites per year.”
This seems to be a good application for electron tubes. They’re almost immune to EMPs and radiation like this. Modern battery tubes don’t require much heating, also. Let’s just think of the Nuvistor. :)
I think coarse structured discreet transistors are also not very vulnerable. But they don’t help you much building a more complex CPU or memory. Same with tubes.
Plastic, being wonderfully high in hydrogen atoms yet nice and solid is excellent for shielding against high energy particles. This is established. Also, everything in space is hideously expensive – but only as it has mass. So it doesn’t really matter what shape or how complicated the plastic is.
So use Buzz Lightyear figures as shielding. It would be awesome.
water is your friend – you’ll probably need it more than you’ll need plastic
Or there’s the option used on a recent space farce: spread the waste management system throughout the hull. Of course, if you have a malfunction, the proverbial does worse than ‘hit the fan’.
Once we get small fission nuclear reactors on board long term space craft the electromagnetic shielding concept turns from on-paper feasible mission concept (which NASA has already planned before: http://www.spacesafetymagazine.com/space-hazards/radiation/superconducting-magnets-protect-spacecrafts-space-radiation/ ) to something deployable.
Should be noted that we also have materials like Demron available that can be sewn into astronauts IVA & EVA suits to provide further shielding from radiation.
Also storing water in the walls of the craft would provide even better protection.
Plus given advancements in nuclear protection drugs like ExRad, Amifostine, Entolimod can help mitigate or encourage DNA repair and free radical reduction in cells.
As for protecting the electronics from radiation, the knowledge is definitely there. Silicon-on-Insulator/Sapphire, Shielded Chips, Redundant systems, guard rings, etc have brought electronics to near immunity in the short term to low level radiation. Long term TID will still be a problem but hopeful additional shielding (active electromag shielding or passive mass shielding) will help.
Additionally rad-hard electronics are catching up with the times too. Its no longer a 10-15 year lag of rad-hard electronics to what’s current on the market. Companies like Vorago are bringing relatively new ARM chips that are rad-hard to market. No longer will you have to be stuck with ancient architectures like PowerPC!
Honestly I think mankind is much better prepared for mitigating/protecting against radiation for long term spaceflight. Its just a matter of running a couple demonstration missions.
Does the Earth magnetic field deflect cosmic rays? They are incredibly energetic. Solar wind is easy, but a magnet in a ship to protect against hard radiation? And gamma rays zoom right through. I think you have to use ion drives and go slow with the crew living in the center of a big water tank. Or water tank and “oil” tank of useful hydrocarbons.
Agreed, Gamma Rays/X-Rays can’t be deflected by a magnetic field (ship, Earth or otherwise) however most of astronauts radiation dose comes from protons, heavy ions from the Solar Wind and then trapped protons/electrons in the Van Allen belts.
Interestingly if the radiation particle/photon is sufficiently high enough energy it’ll just pass through astronauts/the-ship without much interaction (read: radiation absorption). So it’s really the lower energy to medium energy radiation that’s makes up the bulk of an astronauts absorption. Of course this energy absorption threshold varies wildly due to the target material in question, how much secondary/Bremsstrahlung radiation is produced that’s lower energy, etc etc.
At the end of the day, yeah photons can only be stopped by mass.
If you’re interested, I’d recommend this article. It goes deeply into the maths for radiation shielding in regards to the Apollo missions. Really great read: https://web.archive.org/web/20171112233229/http://www.braeunig.us/apollo/VABraddose.htm
It will be a long time before space travel is safe enough that a trip to Mars increases the chance that cancer is what you die of.
This is an animation of data taken by a radiation detector inside the ISS. You can see the increase in particle tracks as the ISS moves through the South Atlantic Anomaly.
https://youtu.be/M2PIDUKT6qE
Is there any way to use solar maximum gamma ray flares as a power source as negative energy